Forget About the Trap
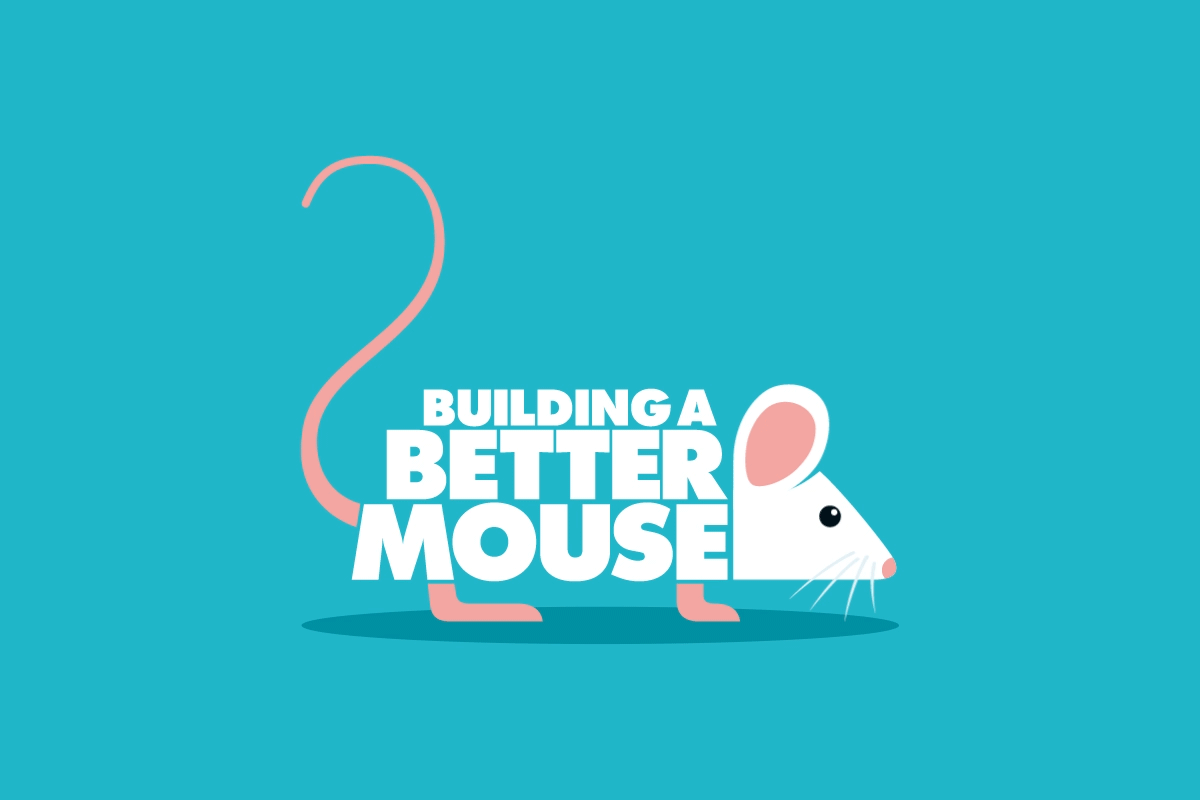
Mice have brains a fraction of the size of human brains. They scamper around on four feet and live just one or two years. But they’re a lot more like us than we realize.
In fact, mice have many of the same genes as humans and can develop the same diseases we are susceptible to, like Alzheimer’s, hypertension, influenza, and various cancers.
Medical researchers use mice to model how diseases can be treated in humans. Researchers have the ability to formulate “mouse models”—that is, representations of diseases via the DNA of mice—to study human diseases.
Why are mice so beneficial to the study of human disease? They are cost effective, easy to house, and breed rapidly, making it convenient to observe many mice over a short period of time.
And because they share more than 95% of our DNA, researchers can modify the DNA of mice to mimic the effects of certain human diseases, bringing them closer to a potential treatment or cure.
Sometimes, regular mice won’t do for a specific experiment: special mice are called for, with certain traits or attributes.
Genetically engineered mice are actually quite common—and valuable. For example, to study COVID-19 in mice, the mice must have a humanized ACE2 gene—the molecule that allows coronaviruses in. (see sidebar).
At Emory, several researchers have modified mice to further understand the inner workings of human disease and even the human brain.
Take, for example, a mouse model of depression. Depressed mice—or, at least, mice that have been bred over several generations to exhibit depressed behavior—act in ways that are remarkably similar to clinically depressed people. They isolate. They move more slowly. They sit in a corner of the cage instead of exploring. They aren’t as quick at cognitive tasks. They show little interest in food or sex.
Some Emory researchers study depression itself with these mice models, while others come at it through corresponding disorders or emotions—fear, anxiety, post-traumatic stress, inflammation, heart disease, epilepsy. Special populations with depression are being considered as well, including children, teenagers, veterans, new mothers, octogenarians, and adults who were abused as children.
And it’s not only depression. Some researchers study infectious disease or the efficacy of vaccines in mice. Others study neurological disorders, like ALS or dystonia or epilepsy. Still others study the microbiome of mice raised in completely sterile environments.
The following is just a sampling of the important work Emory researchers are doing with mouse models. The mice, says one, are “outstanding at what they do.” So here’s to the mice—and to the humans whose lives they save and improve.
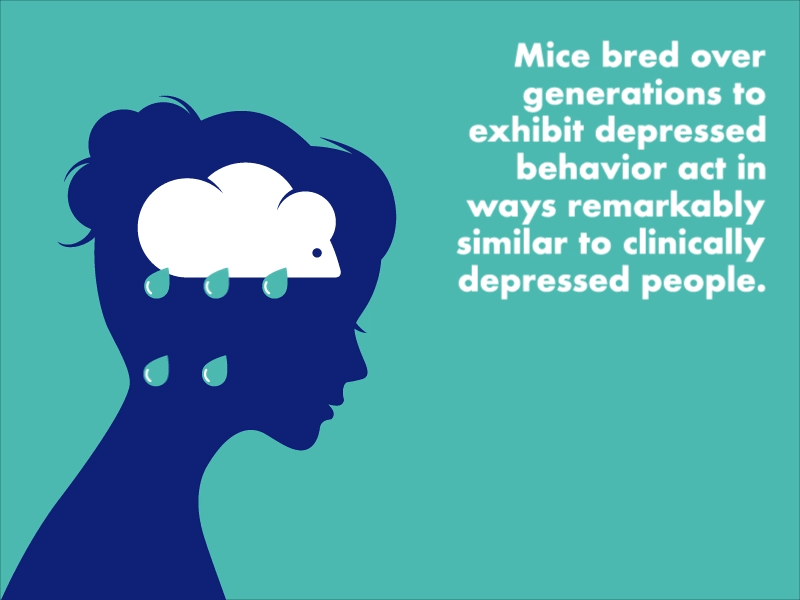
Of Mice and Muscles
Ellen Hess, a professor in the departments of pharmacology and chemical biology and neurology at Emory’s School of Medicine, studies dystonia in mice. Dystonia, a disorder where the muscles of the body contract involuntarily, can cause a person’s body to twist into unnatural positions and often results in pain.
Hess says dystonia is a “defect in communication between brain regions” — and since it is not a degenerative disorder causing the deterioration of neurons, the possibility of returning the brain regions to their normal-functioning abilities does not seem too far off.
“We can use mouse models of dystonia to understand how the problem starts, what is going wrong in the brain to cause dystonia, and to discover new treatments,” Hess says.
Using a mouse model of DOPA-responsive dystonia (DRD)—a term for multiple types of dystonia— Hess can study how brain defects lead to the disorder and search for new forms of therapy to treat it.
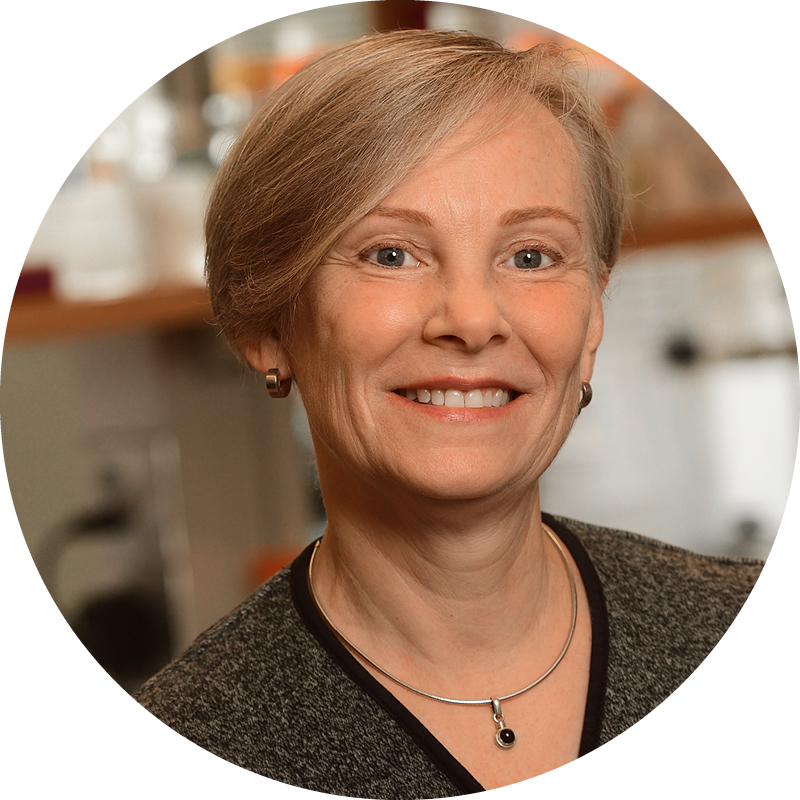
Ellen Hess is a professor of pharmacology and neurology.
“DRD mice recapitulate the human disorder from genes to behavior, making them very useful for understanding the brain defects that give rise to dystonia and for the development of therapeutic strategies,” Hess says.
To successfully model DRD in mice, the mice must have the gene defect that causes DRD in humans. In creating these “knock in” (or KI) mice, Hess and her team must insert new gene sequences into the mice, effectively allowing them to develop dystonia.
These modified mice are not simply bodies to house dystonia — they have also contributed to the discovery of a fundamental aspect of DRD.
Researchers in the past have debated which regions of the brain are involved in dystonia. “This is surprising,” Hess says, “because the brain regions involved are fundamental to understanding a neurological disorder.”
In studying their modified mice, Hess and her team have discovered that dystonia can stem from different brain regions or miscommunication between the basal ganglia and cerebellum.
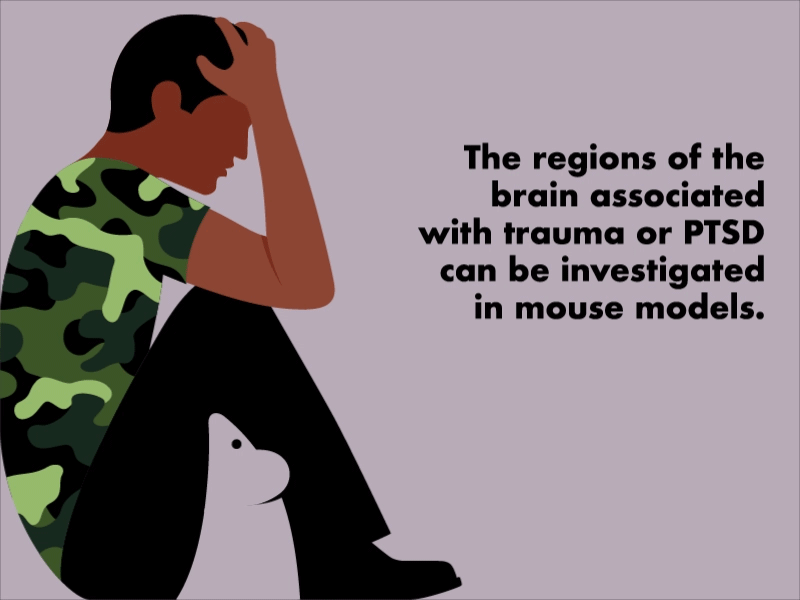
Fear Factor
Brian Dias is no stranger to working with animals. “I’ve worked with rats, birds, fruit flies, lizards, and now mice,” he says. “Due to wanting to describe biological phenomena from a causal perspective, I’ve always done work with a variety of species,” he says.
Dias, a researcher at Yerkes National Primate Research Center and an assistant professor of psychiatry at the School of Medicine, specifically studies fear in mice. In fact, one of his most recognized research findings is that a fear of certain smells can be trained into one generation of mice and passed down to the next generation without direct conditioning of the younger mice, a phenomenon known as “epigenetic inheritance."
Brian Dias is an assistant professor of psychiatry.
Using chemogenetics and optogenetics, two techniques that allow researchers to understand the relationship between brain activity and matter, Dias can inject animals with drugs that will either activate or inhibit the firing of cell populations in their brains.
In doing so, Dias and his team can model human fears like PTSD or anxiety in these mice — and delve deeper into unfamiliar regions of the brain that may be associated with trauma or stress-related conditions.
In particular, Dias considers the zona incerta, a region of gray matter in the brain likely linked with pain that “modulates fear-related behaviors.”
“When we need to look at [PTSD or anxiety], we’ve always traditionally focused on a few regions,” Dias says, citing the amygdala, hippocampus, and prefrontal cortex as major regions in the study of trauma. “Now, with new technologies, we are able to investigate brain regions we knew little about and broaden the neuro landscape. It points us in new directions that allow us to eventually come up with better therapeutic outcomes.”
Dangers of Adolescence
Shannon Gourley’s research is concentrated in behavioral neuroscience.
An associate professor in the Department of Pediatrics at the School of Medicine, Gourley is interested in issues of depression and addiction — specifically, why adolescent humans are more vulnerable to depression and addiction than humans in other stages of life.
Using mice, Gourley can begin to tackle this complicated problem. Like humans, mice also have an adolescence period, although this period lasts for a much shorter time in mice.
“We can study adolescent mice in a month,” Gourley says, which makes them the ideal candidate for her research. She adds that researchers “can ask mice to make very complex decisions.”
In observing mice—adolescent and adult—making these complex decisions, Gourley and her team can observe the differences and investigate how certain genes impact decision-making behaviors that can lead to depression or addiction.
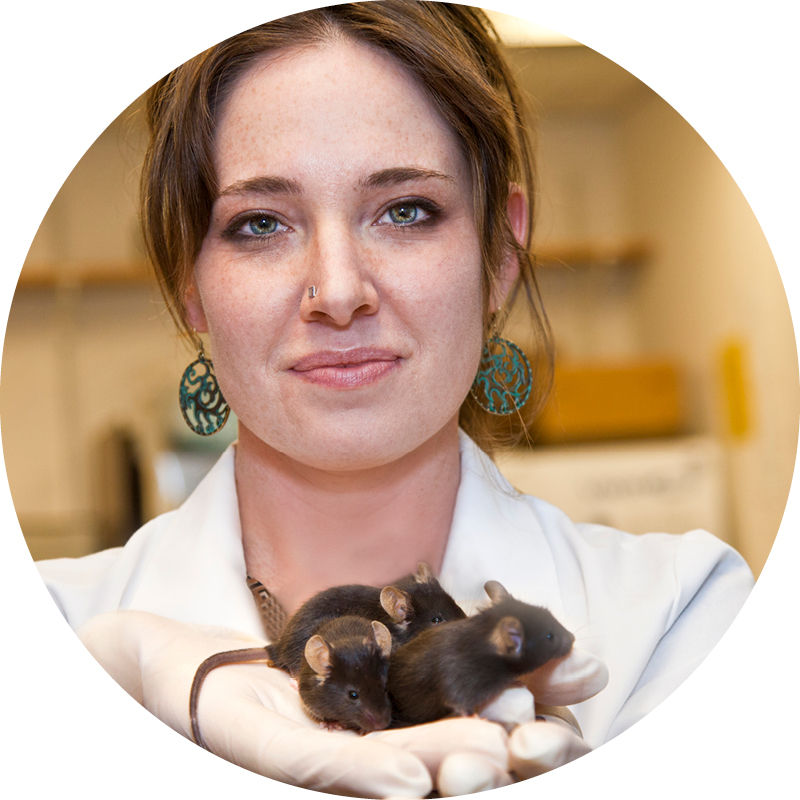
Shannon Gourley is an associate professor of pediatrics.
Such observations could lead to a pivotal finding: a step toward developing better antidepressants. “Classic antidepressants are effective for about half the population,” Gourley says. “That’s not very good.”
But new evidence in this struggle to create more effective antidepressants has arisen. As it turns out, exposing an animal to prolonged stressors can actually change the shape of neurons in the brain. As a result, some neurons in the brain will grow and others will shrink.
“We know that neuron shape changes occur in the case of adolescent stressor exposure, and that these changes can be really persistent even after the stress has ended,” Gourley says. “Perhaps, if we instead make chemicals that act on the building blocks of the neuron structure, we might be able to create new antidepressant strategies that are better.”
With the help of some mice, her team set out to test whether drugs that act on the proteins that influence neuron shape can have antidepressant-like properties–and found one compound that can.
“We published that literature,” says Gourley, who hopes this discovery will lead to clinical trials for these compounds.
The mice in Gourley’s research, she says, are outstanding in what they do.
“I think it’s pretty amazing that, if we are clever enough, we can design tasks that allow our mice to speak to us without words,” she says. “Using the mouse as a model, we can begin to understand the actual neuro changes that are occurring as a result of problem solving. The mice can show us through their actions how they solve complex problems.”
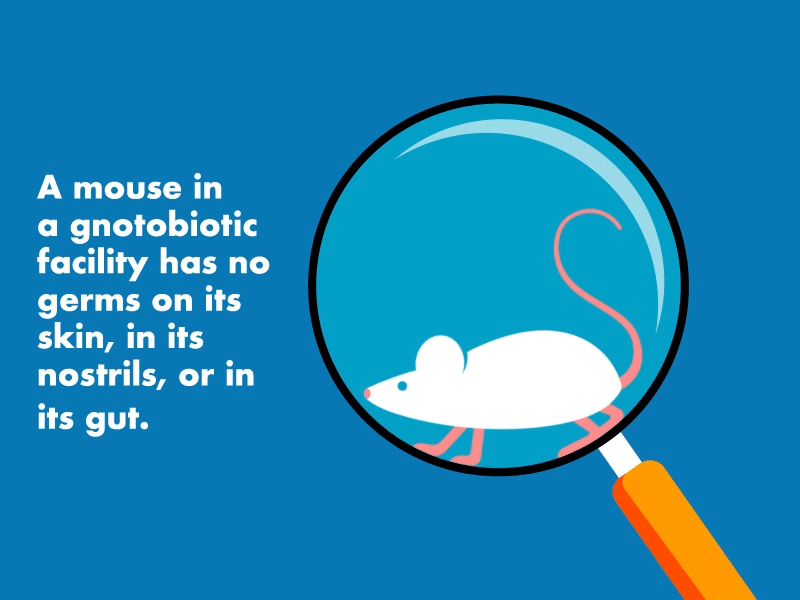
Sterile Mice (and not in the way you think)
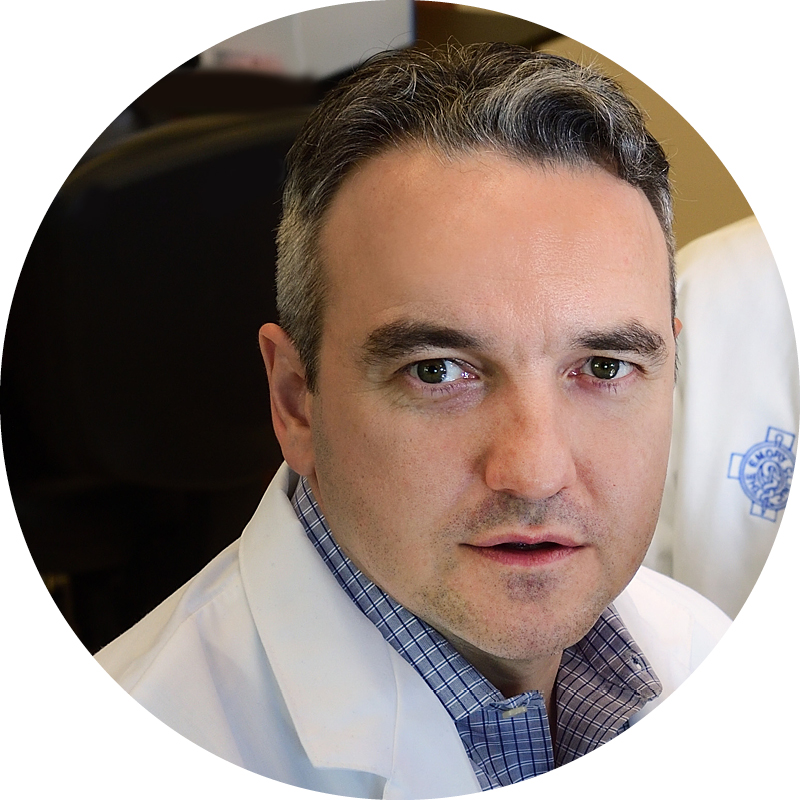
Rheinallt Jones is an assistant professor of pediatrics.
Emory’s gnotobiotic mouse facility was developed by gastroenterologist Rheinallt Jones, assistant professor of pediatrics at Emory School of Medicine, for his studies into the microbiome's influence on host physiology.
“My research includes discovering the molecular mechanisms whereby beneficial bacteria known as ‘probiotics’ elicit their positive influences on health and disease,” Jones says.
Emory’s Gnotobiotic Animal Core offers investigators the opportunity to experimentally manipulate the microbiomes of mice in a controlled environment to gain insight into important biological mechanisms.
The average mouse, like the average human, is home to trillions of bacteria and viruses. But a mouse in a gnotobiotic facility has no germs on its skin, in its nostrils, or in its gut.
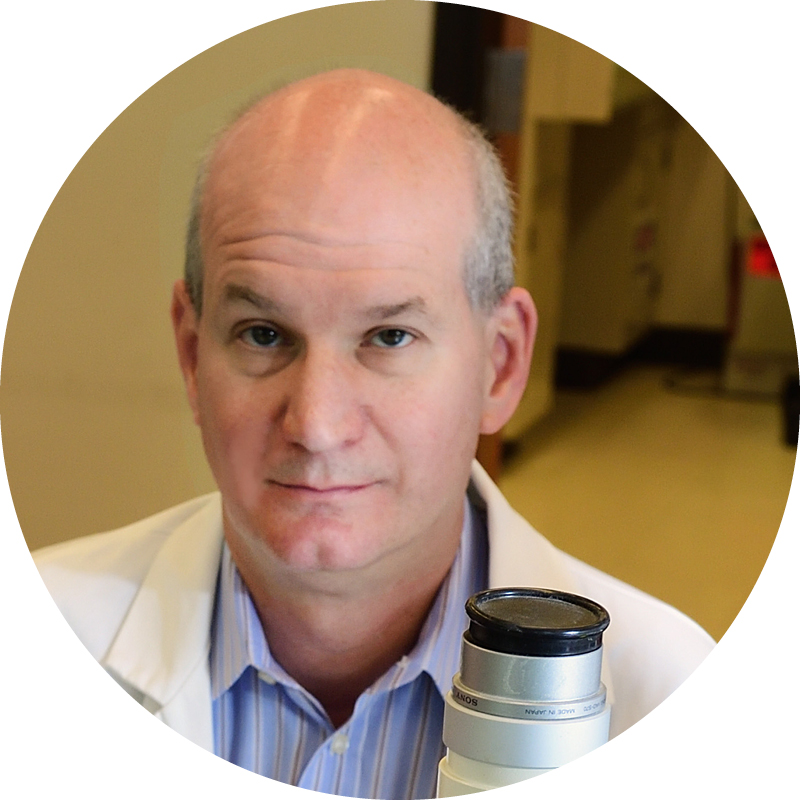
Andrew Neish is a professor of pathology and laboratory medicine.
Its food, water, and bedding is heated to more than 100°C to kill bacteria and viruses before being delivered to the mouse's cage through a sterile system.
“This facility provides a germ-free environment, so you can study the biology of whole organisms with a complete absence of bacteria,” says pathologist Andrew Neish, professor of pathology and laboratory medicine. His research looks at how bacteria contribute to intestinal epithelial integrity and healing.
In the gnotobiotic facility, the microbiome of test animals can be manipulated and defined by researchers.
For example, researchers have used these mice to study the microbiome's influence on neural development: in other words, the gut's influence on the brain.
The more we discover from mice and their interactions with the environment, the more we learn, in turn, about ourselves.
Email the Editor